Rosmarinic acid (RosA) is an ester of caffeic acid and 3,4-dihydroxyphenylactic acid. RosA has a number of beneficial biological activities, including antiviral, antibacterial, anti-inflammatory, and antioxidant properties. Oxidative stress is recognized as a common factor in many neurodegenerative diseases and is a proposed mechanism for age-related degenerative processes as a whole. RosA has shown antioxidative action, acting as a scavenger of free radicals. RosA protects neurons from oxidative stress, significantly attenuated hydrogen peroxide-induced reactive oxygen species generation, and apoptotic cell death and could contribute at least in part to neuroprotective effects. RosA has also been shown to suppress immunoglobulin responses and inflammatory responses of polymorphonuclear leukocytes which may explain its effectiveness at treating seasonal allergic rhinoconjuctivitis in human clinical trials. The anti-inflammatory effects of RosA along with its immunomodulatory effects may also make it a novel agent for the treatment of auto-immune disorders. This review is focused on the effect of RosA on both immunological and neurological systems and its potential as a novel therapeutic agent for the treatment of associated conditions, especially those in which oxidative stress is a factor.
Rosmarinic acid (RosA) is an ester of caffeic acid and 3,4-dihydroxyphenylactic acid (Figure 1). RosA is most commonly found in species of the Boraginaceae and the subfamily Nepetoideae of the Lamiaceae.1 However, it is also found in species of other higher plant families and in some fern and hornwort species.1 RosA has been reported to have beneficial biological activities, including antiviral, antibacterial, anti-inflammatory, and antioxidant properties.1
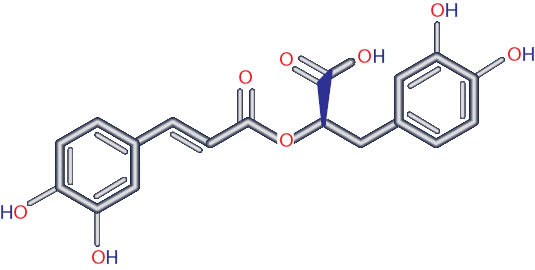
Figure 1Structure of rosmarinic acid.1
Many of these plant species have been used in traditional medicines for a wide variety of conditions including infections, exhaustion, weakness, inflammation, and memory enhancement.2A number of these species, including Lycopus virginicus, Lycopus europaeus, and Melissa officinalis also have a history of treating thyroid conditions, specifically hyperthyroidism.3
Upon ingestion, RosA is found systemically in its intact form, and also as various metabolites such as m-coumaric acid, m-phenylhydroxypropionic acid, and sulfated forms of caffeic, coumaric, and ferulic acids.4
Ingestion of Perilla extract containing 200 mg RosA while fasted results in a peak concentration of approximately 1.15±0.28 μmol/L in the plasma after 30 minutes, and the methylated metabolite (methyl-rosmarinic acid) may rise to a peak of 0.65±0.07 μmol/L at the 2 hour mark.5 Up to 75% of RosA and its metabolites are excreted within the first 6 hours after ingestion.5
RosA has also been shown to be absorbed through the skin in a rat model. The accumulation of RosA favors skin, muscle, and bone deposition rather than organ deposition percutaneously.6 The absolute bioavailability studied in this manner is 60%, which is enhanced by ethanol solvent; a dose of 3 mg over 20 cm2 was used in this model.6
INFLAMMATION
Inflammation underlies a wide variety of physiological and pathological processes.7 At its core, inflammation is an adaptive pathophysiological response to noxious stimuli, including tissue damage and infection.7 The most notable effect that RosA has on inflammation is in its interaction with the complement pathway. Activation of the complement pathway mediates many factors of the inflammatory process including chemotaxis, neutrophil activation, histamine release, as well as upstream signaling for prostacyclin biosynthesis.8–10 RosA has been shown to inhibit complement both in vivo and in vitro.9 Evidence shows that RosA predominantly inhibits complement activation by covalently reacting with the activated compliment component C3b.10 What makes RosA unique as an anti-inflammatory agent is that its mode of action differs from other modulators of complement, namely non-steroidal anti-inflammatory drugs (NSAIDs) and glucocorticoids. RosA does not interfere with cyclooxygenase, rather it inhibits prostanoid release at the site of inflammation where complement activation is taking place.8 NSAIDs and glucocorticoids may cause side effects owing to their systemic inhibition of prostanoid release, with known effects on gastrointestinal health, kidney function, and platelet aggregation. These negative effects may be mediated with RosA as they would only occur at the site where compliment is activated.8
ANTIVIRAL PROPERTIES
RosA also possesses antiviral properties alongside its anti-inflammatory properties which makes it potentially useful in specific clinical scenarios. Swarup et al. investigated the effects of RosA in a murine model of Japanese encephalitis (JEV).11 RosA treatment following JEV infection reduced the mortality rate to 20% (12 out of 15 animals survived following RosA treatment of the JEV-infected and RosA-treated group).11 RosA dramatically reduced the levels of pro-inflammatory cytokines and chemokines. The levels of interleukin (IL)-12, tumor necrosis factor (TNF)-α, g-interferon and IL-6 were significantly decreased in the infected animals treated with RosA compared with the levels in infected animals without treatment.11 In the brains of the JEV-infected group, star-shaped activated microglia appeared more frequently (by more than 30-fold) than in the brains of the control group. RosA treatment completely eliminated the expression of viral proteins and significantly reduced viral mRNA transcripts.11
EFFECTS ON T CELL PATHWAYS
RosA has been shown to modulate T antigen cell receptor (TCR) pathways. TCR mediated signaling has been identified as one of the mechanisms of a number of anti-inflammatory drugs including NSAIDs and anti-rheumatic drugs.12 One particular study explored RosA effects on bone marrow derived dendritic cells (BMDCs).12 This is of particular interest because BMDCs play key roles in T cell priming, Th1/Th2 responses, and can secrete a variety of inflammatory chemokines that can contribute to the pathology of a number of inflammatory conditions.13 It was shown that RosA inhibits the phenotypic maturation and migration of BMDCs, and that it also down-regulates monocyte chemoattractant protein-1 (MCP-1) and macrophage inflammatory protein-1α (MIP-1α) gene expression and consequently significantly inhibits the mitogen-induced protein kinases (MAPK) as well as nuclear factor-kappaB (NF-kB) signal pathways.13 These MAPK and NF-kB signaling pathways are important to the phenotype maturation and migration of BMDCs and are essentially required for chemokine expression.12,13
Cellular apoptosis is essential for normal lymphocyte development and homeostasis in the immune system.14 T cell apoptosis inducers have great potential for the treatment of autoimmune diseases and allograft rejection.15,16 Many drugs are currently used for rheumatoid arthritis and allograft transplantation, including methotrexate and sulfasalazine. These pharmaceutical agents have been shown to kill leukocytes, including T cells, macrophages, and monocytes.15,16 Inducing apoptosis of lymphocytes in order to eliminate persistent or undesired immune responses is of significant interest in pharmaceutical research particularly in the area of allograft transplantation and autoimmune diseases.17 Frequently, more than one mechanism is involved in drug-induced immunosuppression, and apoptosis is recognized as one of these mechanisms.17
In multiple studies conducted by the same group it was shown that RosA can induce T cell apoptosis.17,18 The initial study found that RosA induced apoptosis of Jurkat T cells and investigated the potential pathways of this activity.17 Investigating peripheral blood cells from normal healthy donors indicated that RosA induced apoptosis in T and NK cells in an Lck-dependent manner, but not in Lck-deficient B cells and monocytes.17 Lck is predominantly expressed in T and NK cells and plays an important role in TCR-induced signal transduction.17 Furthermore, actively proliferating T cells were more prone to RosA-induced apoptosis than were resting T cells.17 RosA likely induces apoptosis by eliciting mitochondrial dysfunction with Lck potentially playing a role in this pathway.17
This was expanded on and confirmed in a follow up study. This study investigated the effects of RosA on T cells from rheumatoid arthritis patients and further explored the T cell populations that were targeted by RosA.18 It was demonstrated that the major target population of RosA-induced apoptosis included the activated T cell subsets, namely CD3+CD25+ and CD4+CD25+ T cells.18 RosA-induced apoptosis of CD3+CD25+ activated T cells from 57% of rheumatoid arthritis patients and its effect was dose-dependent.18 RosA preferentially induced apoptosis of activated T-cell subsets over resting T cells, as was demonstrated in the previous study.17, 18 More interestingly, RosA was observed to have more potent apoptotic activity on CD4+CD45RO+ effector T-cell subsets than on CD4+CD45RosA+ naive T-cell subsets.18 CD4+ effector/memory T cells are the major T-cell populations observed in the synovium of rheumatoid arthritis patients.18 CD4+CD45RO+ subsets from rheumatoid arthritis patients have been shown to be resistant to induced apoptosis.18 This resistance of potentially self-reactive T cells to activation-induced cell death or drug-induced apoptosis has been suggested to be one of the causes of rheumatoid arthritis pathogenesis.18 The apoptotic activity of RosA on potentially pathogenic CD4+CD45RO+ effector T cells may be very beneficial for rheumatoid arthritis patients.18 Because RosA preferentially induced apoptosis of activated T cells from healthy volunteers in the previous study,17 RosA-induced apoptosis may not be limited to T cells from rheumatoid arthritis patients, but may show benefits in other populations as well.18
In an animal model of collagen-induced arthritis it was shown that RosA dramatically reduced the arthritic index score and the number of affected paws in treated mice.19 Histopathologic observations confirmed this, showing that RosA-treated mice retained near normal architecture of synovial tissues, whereas control mice displayed severe synovitis.19 The histopathologic index was scored according to the degree of leukocyte infiltration and bone invasion. The mean histopathologic index of control mice was 3.31±0.25. It was seen manifested by severe leukocyte infiltration and loss of bone integrity found the majority of the mice.
In comparison, the joints of RosA injected mice were predominantly normal, with few instances of early arthritis in some mice, as evidenced by a lower mean histopathologic index of 0.60±0.21 (P<0.0001). Synovial tissues from RosA-treated mice exhibited a considerably reduced frequency of COX-2-expressing cells, compared with those from untreated mice.19 What is more interesting to note is that the researchers found that RosA inhibits T cell receptor-mediated T cell activation and proliferation, which further implies the immunosuppressive potential of RosA in T cell-mediated immune disorders.19 T cells obtained from RosA-treated mice exhibited a decrease in the proliferative response upon in vitro stimulation with type 2 collagen.19 The authors conclude that RosA may provide a therapeutic effect to treat rheumatoid arthritis in a clinical setting.19
EFFECTS ON ALLERGIC RESPONSE
A number of studies have looked at the effects of RosA on allergies, namely allergic rhinitis/rhinoconjunctivitis. Allergic rhinitis/rhinoconjunctivitis is characterized by a two-phase allergic reaction. In the early phase, immunoglobulin E (IgE) dependent activation of mast cells and basophils results in the production of inflammatory mediators including histamine, prostaglandins, leukotrienes and cytokines which produce sneezing, rhinorrhea, and pruritis.20 The late phase of allergic rhinitis/rhinoconjunctivitis is characterized by the accumulation of mast cells, eosinophils, and basophils in the epithelium.20 Recruitment of inflammatory cells, including eosinophils, basophils, and T cells, leads to further releases of histamine, leukotrienes, and other compounds including pro-inflammatory cytokines, cyclooxygenase-2, and chemokines, which all act in unison to sustain the allergic response.20,21
These findings on the anti-inflammatory and immunological effects of RosA likely explain the compelling results seen in both animal and human trials. In a study by Sanbogni et al., in which mice were exposed to a dust mite allergen in order to induce an allergic response, mice that were given RosA in perilla extract at a dose of 1.5 mg/day, showed an inhibition of mucus cell accumulation as well as inhibition of eosinophilic inflammation in the lung (P<0.05).22 More importantly, it was observed that RosA in perilla extract decreased production of allergy-induced IL-4 and IL-5, significantly inhibited Th2 cytokines and chemokines as well as significantly inhibited of allergen specific IgG (P<0.05).22
Another study looked at both in vitro and in vivo models to examine the effects of RosA on allergic inflammatory responses.23 In mice it was seen that levels of IgE, histamine, IL-1b, IL-6, and TNF-α were reduced by RosA administration (P<0.05).23 In the RosA-treated mice, the mast cell and eosinophil infiltration was reduced in the nasal mucosa and lower levels of cyclooxygenase-2 were observed.23 In activated human mast cells, NF-kB was inhibited after a treatment of RosA.23 These results indicate that RosA may ameliorate allergic inflammatory reactions such as allergic rhinitis and allergic rhinoconjunctivitis.23
Human studies have had similar results as the animal models. In a 21-day, randomized, double blind, age matched, placebo controlled, parallel group study, 30 participants with seasonal allergic rhinoconjunctivitis received either a low (50 mg) or high (200 mg) dose of Perilla extract enriched with RosA vs placebo.24 Symptoms were recorded and tracked, levels of inflammatory markers collected from nasal lavage, and serum IgE levels were measured as well. A dose-dependent decrease in overall symptoms was observed at the end of the trial. Overall symptom relief was reported as follows: 55.6% of the low dose group and 70% of the high dose group, compared with 30% of the placebo group.24 Compared with placebo, oral supplementation of RosA (50 or 200 mg) resulted in significant increases in responder rates that were based on symptom score reductions on Day 21 (P<0.05). The 50-mg dose significantly increased the responder rate on the total symptom score on Day 3 (P<0.05 vs. placebo). The responder rates for the total symptom score were also greater in the 200 mg group than in the placebo group on Days 3 and 21, although these increases did not achieve statistical significance. There was a significant reduction in immune cells in the nasal mucus by the third day in the high dose group [polymorphonuclear leukocytes reduced by 84%, eosinophils reduced by 86%, neutrophils reduced by 72% (P<0.05 vs. placebo)] but this effect appeared to normalize, showing no significant difference between the groups at day 21.24 No adverse effects were reported and no significant blood abnormalities (complete blood cell counts, hepatic and renal function tests, and creatine phosphokinase) were found suggesting that RosA is a safe and viable treatment option for seasonal allergic rhinoconjunctivitis.24
NEUROLOGICAL DISEASE AND OXIDATIVE STRESS
There are a wide variety of neurodegenerative diseases with distinct symptoms and pathologies. Oxidative stress is recognized as a common factor in many neurodegenerative diseases and is a proposed mechanism for age-related degenerative processes as a whole.25 Numerous studies have provided compelling evidence linking neuronal oxidative stress to Parkinson’s disease (PD), Alzheimer’s disease (AD), amyotrophic lateral sclerosis (ALS), and multiple sclerosis (MS), to highlight but a few.25 Although several protective antioxidant molecules in the brain such as superoxide dismutase (SOD), glutathione peroxidase and ascorbate can remove the reactive oxygen species (ROS), oxidative stress is caused by the imbalance between the rate of oxidant generation production and the level of antioxidants present.26 Based on the premise that oxidative stress underlies a number of neurodegenerative diseases, the identification of novel antioxidants as potential therapeutics is a fertile area of neuroscience research.25
Oxidative stress has been implicated in causing neuronal cell death.25,26 RosA has been investigated in its effects in the prevention of neuronal cell death. One study evaluated the use of RosA in hydrogen peroxide (H2O2)-induced neurotoxicity in human dopaminergic cells (cell line SH-SY5Y).26 It was observed that pretreatment of the cells with high concentration of RosA almost completely inhibited H2O2-induced cell death.26 It was also observed that RosA upregulates heme oxygenase-1 (HO-1).26 HO-1 is an inducible enzyme with strong antioxidant properties, and the up regulation of this enzyme may be essential for RosA to mitigate H2O2-induced cytotoxicity as well as exert antioxidant effects.26
In another study investigating RosA effects on the same cell line (SH-SY5Y) similar results were found.27 In this study, cells were exposed to tert-butylhydroperoxide for oxidative stress, L-glutamate for excitotoxicity, and oxygen-glucose deprivation/reoxygenation for ischemia–reperfusion.27 Cells were pretreated with either RosA or clovamide, a similar phenolic compound found in cocoa and coffee beans.27 Both RosA and clovamide were effective in preventing neuronal cell death in all three in vitro models of neuronal death: oxidative stress, excitotoxicity, and ischemia–reperfusion.27 What is interesting to note is the RosA, but not clovamide, was able to inhibit NF-κβ translocation and increase peroxisome proliferator-activated receptor-g (PPAR-g) expression in cells that were not exposed to damaging stimuli.27 This is an important finding as necrotic cells release factors which can induce a cascade of pro-inflammatory events causing damage to neighboring tissues. This cascade can be inhibited by PPAR-g and antagonized by NF-κβ.27
In a study using a mouse model of amyotrophic lateral sclerosis (ALS), RosA was shown to have significant benefits.28 Mice were treated with rosemary extract, RosA, or carnosic acid, intraperitoneally administered at a dose of 3, 0.13, and 0.13 mg/kg, respectively.28 The study found that both rosemary extract and RosA extended the overall survival of ALS model mice and effectively improved the impairment of motor performance attributed to ALS disease progression.28 Additionally, both rosemary extract and RosA were found to attenuate the degeneration of motor neurons.28 However, RosA, alone, imparted a clear improvement in the clinical scoring test and resulted in significant suppression of body weight loss relative to the vehicle control group (P<0.05), whereas rosemary extract alone showed no such benefits.28 This study suggests that RosA is one of the key molecules in rosemary extract that exerts a neuroprotective effect, and individually may offer additional benefits.28
EFFECTS ON COGNITION
RosA may be able to improve cognition by inhibiting prolyl oligopeptidase (POP). POP is a protease that cleaves arginine-vasopressin (AVP), substance P (SP), oxytocin, and angiotensin IV, which have all been shown to have neurological function in addition to their physiological functions.29 In this study it was shown that RosA is a potent inhibitor of POP, even more so than another known natural POP inhibitor berberine.29 In this same study it was shown that these effects were associated with improved cognition in rats who received either acute or sub-chronic doses of RosA.29 Spatial memory was measured using the Morris water maze task. Swimming behaviors were recorded by video camera and analyzed using an automated tracking system, for path length, time spent in the target quadrant, the number of platform crossings, and speed.29 Subchronic treatment of RosA significantly increased the number of crossings, in particular at 2 mg/kg dose. Although the time spent in the target quadrant of the 2 mg/kg treated group was not significantly greater than that of vehicle-treated controls, the number of crossings was significantly increased as compared to controls (2 weeks, P<0.05; 3 weeks, P<0.05). Because the number of crossings can be used to measure spatial memory performance, this result indicates that subchronic administration of RosA for 2 or 3 weeks enhances spatial memory. Although the cognitive-enhancing effect of RosA showed a U-shaped response curve29 (losing effects at higher and lower doses) this can be explained as many several endogenous peptides are known to show the same paradoxical decline of cognitive-enhancing effects at higher doses.30
Alzheimer’s disease (AD) is the most common form of dementia, resulting in deterioration of cognitive function and behavioral changes.31 One of the pathological hallmarks of AD is extracellular deposits of aggregated amyloid-β protein (Aβ) in the brain parenchyma (senile plaques) and cerebral blood vessels (cerebral amyloid angiopathy).31
ACETYLCHOLINESTERASE INHIBITION
One of the most effective treatments for AD, at present, consists of increasing the levels of acetylcholine through the inhibition of acetylcholinesterase activity.32In vitro studies have shown that extracts of Plectranthus barbatus standardized for RosA have inhibitory effects on acetylcholinesterase.32 A more recent follow up in vivo study by the same group has confirmed the initial findings.33Plectranthus barbatus tea and RosA was administered to rats intragastrically and intraperitoneally.33 Metabolites appearing in the bloodstream and brain were studied in addition to acetylcholinesterase levels in the brain. What is interesting to note is that when RosA was present within the tea extract, a lower quantity of this acid was found in plasma, suggesting that the other extract components may interfere in the gut permeation of RosA.33 When rat brains were analyzed no compounds or metabolites of the tea extract or RosA were found.33 However, when the brain acetylcholinesterase activity was measured, a decrease of 10% and 5.5% of the enzymatic activity was observed, 30 and 60 min after the extract administration, respectively.33 After RosA administration a decrease of 13.5% and 12.8% of acetylcholinesterase activity was observed, at 30 and 60 minutes, respectively.33
EFFECTS ON AMYLOID PLAQUE
RosA as well as other anti-oxidants have anti-amyloidogenic effects.34 RosA dose-dependently inhibited the formation of Aβ as well as destabilizing preformed Aβs.34 It was suggested that a possible mechanism for this is the two 3,4-dihydroxyphenyl rings in the molecular structure of RosA (Figure 1).34 This compact and symmetric structure might be quite suitable for specifically binding to free Aβ and subsequently hindering the polymerization of Aβ.34 Alternatively, this structure might be suitable for specific binding and subsequent destabilization of the β-sheet-rich conformation of Aβ molecules.34
Another study investigated the effects of RosA on amyloid plaques and their consequent memory impairment.35 Peroxynitrite (ONOO−) mediated damage is considered to be responsible for the cognitive dysfunction induced by Aβ protein in AD.35 Mice received intracerebroventricular injections of Aβ and/or ONOO−. RosA prevented the ONOO-induced nitration of proteins and impairment of memory.35 Daily treatment with RosA significantly prevented the nitration of proteins as well as memory impairment induced by Aβ.35 However, RosA protection of AB toxicity was all but eliminated after the co-injection of non-memory impairing dose of proxynitrites to increase the proxynitrite burden.35 RosA did not increase or improve memory function in normal mice.35 It is then said that its memory protecting effects in this case are limited to its antioxidant properties.
SAFETY
In mice RosA was shown to have very low toxicity with an LD50 of 561 mg/kg.1 Further investigation into RosA’s effects on specific organ systems were conducted in mice. A 10, 25, or 50 mg/kg dose of RosA was given orally once daily for 2 days, 6 hours after injection of the hepatoxin carbon tetrachloride.36 RosA did not alter the liver weight of the animals or raise the ALT levels. RosA in fact improved histological and serum markers of liver damage induced in the study.36
Acceptable daily intake (ADI) is a measure of the amount of a specific substance in that can be orally consumed on daily basis, over a lifetime without an appreciable health risk.37 An ADI value can be calculated from the no observed adverse effect level (NOAEL).37 In a study using AD model transgenic mice, RosA exhibited no adverse effect at a level of 1 g/kg in the study.37 Assuming a human weight of 50 kg, NOAEL was determined as 50 g per day.37 The ADI value was then calculated to be 500 mg per day.37
In the human trials conducted, with doses of RosA of up to 500 mg per day, no abnormalities were observed on blood tests which included complete blood cell counts, hepatic and renal function tests, total protein, electrolytes, lipids, uric acid, and creatine phosphokinase.24,37 RosA appears to be safe for human consumption.
DOSING
Dosages used in the studies vary depending on the method of administration but inferences can still be drawn based on the available evidence. When administered by injection the dosages used ranged from 0.1 to 10 mg/kg9,28,35 up to 50 mg/kg19 in animal models. All of these trials showed favorable results but it is of particular interest that the studies with varied doses showed little dose-dependent activity, achieving favorable outcomes with lower doses of RosA.9,35 In particular, it was noted that the optimal dose of RosA that protects against memory impairment was the 0.25 mg/kg.35
In animal studies using oral administration, a similar trend is observed. Dosages in animal models ranged from 1 to 8 mg/kg.22,29 What is of interest in these studies is that the 2-mg/kg dose of RosA produced the most favorable results when compared with the other doses, both higher and lower.29
In human trials the doses used were 50 mg and 200 mg studied for its effect on seasonal allergic rhinoconjunctivitis.24 RosA was effective at both the lower and higher doses without much difference between the two groups in both reported symptoms and laboratory evaluations.24
From the available evidence we see that RosA is safe and effective for human consumption at doses ranging from 50 to 500 mg. The optimal dose of RosA based on the available evidence would be in the low to moderate end of the dosing spectrum as results have shown that the peak physiological effects may be optimal at these lower doses. If we administer an oral dose of 1–2 mg/kg this would translate to 60–120 mg for a 60-kg individual and 100–200 mg for a 100-kg individual. Although higher dosages are likely safe, use of lower doses may prove to be optimal for some conditions, specifically in cases of cognitive impairment. A summary of rosmarinic acid research on immunological effects is listed in Table 1 and on neurological effects in Table 2.
Tablel 1
Summary of rosmarinic acid research on immunological effects.
Summary of rosmarinic acid research on immunological effects.
Study type | Activity | Results/Mechanism | References |
---|---|---|---|
In vivo | Anti-inflammatory | Diminished action of complement C3 and C5 | Rampart et al.8 |
In vitro | Anti-inflammatory | Inhibition of complement C3-convertase | Englberger et al.9 |
In vivo | Anti-inflammatory | Inhibition of complement via attachment to complement C3b | Sahu et al.10 |
In vivo | Anti-inflammatory, Immunomodulatory | Inhibits MAPK and NF-κB pathways, inhibits phenotypic maturation and migration of bone marrow derived dendritic cells | Kim et al.13 |
In vitro | Antiviral, Anti-inflammatory | Reduction in IL-12, TNF-α, γ-interferon and IL-6, increased mortality rates, inhibited expression of viral proteins and reduced viral mRNA transcripts | Swarup et al.11 |
In vivo | Immunomodulatory | Inducement of apoptosis in T and NK cells via eliciting mitochondrial dysfunction | Hur et al.17 |
In vivo | Immunomodulatory | Preferentially induced apoptosis in activated T cell subsets in patients with rheumatoid arthritis | Hur et al.18 |
In vitro | Anti-inflammatory, Immunomodulatory | Reduced arthritic index scores, inhibition of synovial tissue damage, reduced COX-2 expression, and inhibit TCR-mediated T cell activation and proliferation | Youn et al.19 |
In vitro | Anti-inflammatory, Immunomodulatory | Inhibition of mucus cell accumulation, inhibition eosinophilic inflammation, decreased IL-4 and IL-5, inhibition of Th2 cytokines and inhibition of allergen specific IgG | Sanbongi et al.22 |
In vivo and in vitro | Anti-inflammatory, Immunomodulatory | Reduced levels of IgE, histamine, IL-1b, IL-6, TNF-α, reduced mast cell and eosinophil infiltration, reduced levels of cyclooxygenase-2 and NF-kB | Oh et al.23 |
Randomized controlled human trial | Anti-inflammatory, Immunomodulatory | Improvements in global symptom relief (seasonal allergic rhinoconjuctivitis), reduction in polymorphonuclear leukocytes, eosinophils and neutrophils | Takano et al.24 |
IgE, immunoglobulin E; IL, interleukin; MAPK, mitogen-induced protein kinases; NF, nuclear factor; TCR, T antigen cell receptor; TNF, tumor necrosis factor.
Table 2
Summary of rosmarinic acid research on neurological effects.
Study type | Activity | Results/Mechanism | References |
---|---|---|---|
In vivo | Antioxidant, Neuroprotection | Upregulates HO-1, inhibit H2O2-induced cell death | Lee et al.26 |
In vitro | Antioxidant, Neuroprotection | Inhibit cell death under oxidative stress, excitotoxicity, ischemia–reperfusion conditions, inhibit NF-κB translocation and increase PPAR-g expression | Fallarini et al.27 |
In vitro | Neuroprotection | Increase in survival, improved motor performance and reduce bodyweight loss (ALS model) | Shimojo et al.28 |
In vitro | Cognition | Improvement of spatial memory performance | Park et al.29 |
In vitro | Acetylcholinesterase Inhibition | Decrease in acetylcholinesterase activity | Falé et al.33 |
In vivo | Neuroprotection | Inhibited the formation of Aβ as well as destabilizing preformed Aβs | Ono et al.34 |
In vitro | Antioxidant, Neuroprotection | Prevented nitration of proteins and impairment of memory | Alkam et al.35 |
Aβ, amyloid-β protein; ALS, amyotrophic lateral sclerosis; NF, nuclear factor; PPAR-g, peroxisome proliferator-activated receptor-g.
RosA has a number of immunological effects as noted in the above research. In order to attenuate inflammation, compounds that are capable of reducing or eliminating leukotriene production and modulating the inflammatory response without adverse reactions are of great interest. RosA appears to be a promising agent in this regard based on the in vitro and in vivo studies reported. RosA is highly promising in the treatment of auto-immune disorders. Its effects on T cell regulation and inflammation may potentially be of great benefit to treat auto-immune conditions in a clinical setting (Table 1). Human clinical trials have also shown benefit in its use for the treatment and prevention of seasonal allergic rhinoconjuctivitis.
RosA effects on neurodegenerative disease where oxidative stress and immunomodulation are at the underlying cause have shown a potential for its application for use in their treatment. Assessment of its use as a therapeutic agent would be warranted in these disorders. Its effects on cognition, acetylcholinesterase inhibition, and oxidative stress could make RosA a novel agent in the treatment of mild to moderate neurological disorders, such as AD.
The author has nothing to disclose.
- Petersen M,
Simmonds M.
Rosmarinic acid.
- Hajimehdipoor H,
Saeidnia S,
Gohari A,
Hamedani M,
Shekarchi M.
Comparative study of rosmarinic acid content in some plants of Labiatae family.
- Yarnell E,
Abascal K.
Botanical medicine for thyroid regulation.
- Bhatt R,
Mishra N,
Bansal PK.
Phytochemical, pharmacological and pharmacokinetics effects of rosmarinic acid.
- Baba S,
Osakabe N,
Natsume M, et al.
Absorption, metabolism, degradation and urinary excretion of rosmarinic acid after intake of Perilla frutescens extract in humans.
- Ritschel WA,
Starzacher A,
Sabouni A,
Hussain AS,
Koch HP.
Percutaneous absorption of rosmarinic acid in the rat.
- Medzhitov R.
Origin and physiological roles of inflammation.
- Rampart M,
Beetens JR,
Bult H,
Herman AG,
Parnham MJ,
Winkelmann J.
Complement-dependent stimulation of prostacyclin biosynthesis: inhibition by rosmarinic acid.
- Englberger W,
Hadding U,
Etschenberg E, et al.
Rosmarinic acid: A new inhibitor of complement C3-convertase with anti-inflammatory activity.
- Sahu A,
Rawal N,
Pangburn MK.
Inhibition of complement by covalent attachment of rosmarinic acid to activated C3b.
- Swarup V,
Ghosh J,
Ghosh S,
Saxena A,
Basu A.
Antiviral and anti-inflammatory effects of rosmarinic acid in an experimental murine model of Japanese encephalitis.
- Kang M-A.
Rosmarinic acid inhibits Ca2+-dependent pathways of T-cell antigen receptor-mediated signaling by inhibiting the PLC-gamma 1 and Itk activity.
- Kim HK,
Lee JJ,
Lee JS,
Park YM,
Yoon TR.
Rosmarinic acid down-regulates the LPS-induced production of monocyte chemoattractant protein-1 (MCP-1) and macrophage inflammatory protein-1alpha (MIP-1alpha) via the MAPK pathway in bone-marrow derived dendritic cells.
- Rathmell JC,
Thompson CB.
Pathways of apoptosis in lymphocyte development, homeostasis, and disease.
- Genestier L,
Paillot R,
Fournel S,
Ferraro C,
Miossec P,
Revillard JP.
Immunosuppressive properties of methotrexate: apoptosis and clonal deletion of activated peripheral T cells.
- Rodenburg RJT,
Ganga A,
Van Lent PLEM,
Van De Putte LBA,
Van Venrooij WJ.
The antiinflammatory drug sulfasalazine inhibits tumor necrosis factor α expression in macrophages by inducing apoptosis.
- Hur Y-G,
Yun Y,
Won J.
rosmarinic acid induces p56lck-dependent apoptosis in Jurkat and peripheral T cells via mitochondrial pathway independent from Fas/Fas ligand interaction.
- Hur Y-G,
Suh C-H,
Kim S,
Won J.
Rosmarinic Acid Induces Apoptosis of Activated T cells from rheumatoid arthritis patients via mitochondrial pathway.
- Youn J,
Lee KH,
Won J, et al.
Beneficial effects of rosmarinic acid on suppression of collagen induced arthritis.
- Fukui N,
Honda K,
Ito E,
Ishikawa K.
Peroxisome proliferator-activated receptor γ negatively regulates allergic rhinitis in mice.
- Fuentes-Beltrán A,
Montes-Vizuet R,
Valencia-Maqueda E,
Negrete-García MC,
de L. García-Cruz M,
Teran LM.
Chemokine CC-ligand 5 production and eosinophil activation into the upper airways of aspirin-sensitive patients.
- Sanbongi C,
Takano H,
Osakabe N, et al.
Rosmarinic acid in perilla extract inhibits allergic inflammation induced by mite allergen, in a mouse model.
- Oh H-A,
Park C-S,
Ahn H-J,
Park YS,
Kim H-M.
Effect of Perilla frutescens var. acuta Kudo and rosmarinic acid on allergic inflammatory reactions.
- Takano H,
Osakabe N,
Sanbongi C, et al.
Extract of Perilla frutescens enriched for rosmarinic acid, a polyphenolic phytochemical, inhibits seasonal allergic rhinoconjunctivitis in humans.
- Kelsey NA,
Wilkins HM,
Linseman DA.
Nutraceutical antioxidants as novel neuroprotective agents.
- Lee HJ,
Cho H-S,
Park E, et al.
Rosmarinic acid protects human dopaminergic neuronal cells against hydrogen peroxide-induced apoptosis.
- Fallarini S,
Miglio G,
Paoletti T, et al.
Clovamide and rosmarinic acid induce neuroprotective effects in in vitro models of neuronal death.
- Shimojo Y,
Kosaka K,
Noda Y,
Shimizu T,
Shirasawa T.
Effect of rosmarinic acid in motor dysfunction and life span in a mouse model of familial amyotrophic lateral sclerosis.
- Park DH,
Park SJ,
Kim JM,
Jung WY,
Ryu JH.
Subchronic administration of rosmarinic acid, a natural prolyl oligopeptidase inhibitor, enhances cognitive performances.
- Calabrese EJ.
Alzheimer’s disease drugs: an application of the hermetic dose–response model.
- Blennow K,
de Leon MJ,
Zetterberg H.
Alzheimer’s disease.
- Falé PL,
Borges C,
Madeira PAJ, et al.
Rosmarinic acid, scutellarein 4′-methyl ether 7-O-glucuronide and (16S)-coleon E are the main compounds responsible for the antiacetylcholinesterase and antioxidant activity in herbal tea of Plectranthus barbatus (‘falso boldo’).
- Falé PLV,
Madeira PAJ,
Florêncio HM,
Ascensão L,
Serralheiro MLM.
Function of Plectranthus barbatus herbal tea as neuronal acetylcholinesterase inhibitor.
- Ono K,
Hamaguchi T,
Naiki H,
Yamada M.
Anti-amyloidogenic effects of antioxidants: implications for the prevention and therapeutics of Alzheimer’s disease.
- Alkam T,
Nitta A,
Mizoguchi H,
Itoh A,
Nabeshima T.
A natural scavenger of peroxynitrites, rosmarinic acid, protects against impairment of memory induced by Aβ25–35.
- Domitrović R,
Škoda M,
Vasiljev Marchesi V,
Cvijanović O,
Pernjak Pugel E,
Štefan M.
Rosmarinic acid ameliorates acute liver damage and fibrogenesis in carbon tetrachloride-intoxicated mice.
- Noguchi-Shinohara M,
Ono K,
Hamaguchi T, et al.
Pharmacokinetics, safety and tolerability of Melissa officinalis extract which contained rosmarinic acid in healthy individuals: a randomized controlled trial.